Optomechanical cooling with coherent and squeezed light and frequency-dependent mirrors: The thermodynamic cost of opening the heat valve
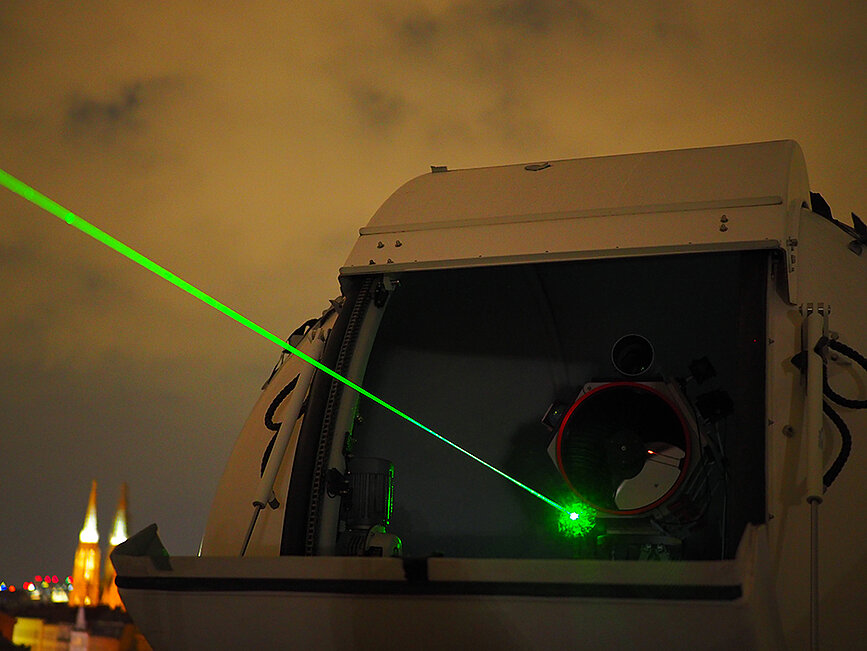
This furthermore makes them promising platforms for exploring stochastic and quantum thermodynamics at the nanoscale, as the mechanical resonator is coupled to a thermal heat bath and, additionally, to an engineerable optical bath. Cooling is a ubiquitous process in thermodynamics, and, in the context of optomechanics, it is employed to bring the mechanical mode close to its ground state, which is a necessary step for the implementation of most optomechanics-based quantum technologies. In cavity optomechanics, such ground-state cooling can be achieved by driving the optical cavity with a coherent laser in the resolved-sideband regime [1].
I will first present a thermodynamic performance analysis of this optomechanical sideband cooling scheme in terms of a heat valve [2]. As performance quantifiers, we examine not only the lowest reachable effective temperature (phonon number) but also the evacuated-heat flow as an equivalent to the cooling power of a standard refrigerator, as well as appropriate thermodynamic efficiencies, which all can be experimentally inferred from measurements of the cavity output light field. Importantly, we investigate two recent alternative schemes for ground-state cooling: using a cavity with a frequency-dependent mirror (Fano mirror) [3] and replacing the coherent laser drive with squeezed light [4]. We study the dynamics of these setups within and beyond the weak-coupling limit and give concrete examples based on parameters of existing experimental systems. By applying our thermodynamic framework, we gain detailed insights into these three different optomechanical cooling setups, allowing a comprehensive understanding of the thermodynamic mechanisms at play.
Secondly, I will focus on the setup of the cooling second scheme, a cavity with a frequency-dependent mirror. I will discuss a generalization of the model from Ref. [3], adding a dissipative optomechanical coupling arising from the change in the loss rate of the cavity due to the mechanical motion. Our model describes better recent experimental results [5], in particular, the mechanical frequency shift. The experimental device, a chip-based microcavity made of a photonic crystal suspended above a distributed Bragg reflector, is especially promising as we find it in the single-photon ultrastrong coupling regime.
[1] M. Aspelmeyer, T. J. Kippenberg, F. Marquardt, Rev. Mod. Phys. 86, 1391 (2014)
[2] J. Monsel, N. Dashti, S. K. Manjeshwar, J. Eriksson, H. Ernbrink, E. Olsson, E. Torneus, W. Wieczorek, J. Splettstoesser Phys. Rev. A 103, 063519 (2021)
[3] O. Černotík, A. Dantan, C. Genes, Phys. Rev. Lett. 122, 243601 (2019)
[4] M. Asjad, S. Zippilli, D. Vitali, Phys. Rev. A94, 0518012 (2016)
[5] S. K. Manjeshwar, A. Ciers, J. Monsel, H. Pfeifer, C. Peralle, S. M. Wang, P. Tassin, W. Wieczorek arXiv:2305.13511
Information
Speaker: Juliette Monsel (Janine Splettstoesser Group, Chalmers University of Technology, Gothenburg)